Carbon nanotubes can conduct electricity due to their unique hexagonal lattice structure derived from graphene.
The sp2 hybridized carbon atoms in this structure create a continuous π-bonding network, allowing for the efficient movement of electrons.
Additionally, carbon nanotubes can exhibit metallic or semiconducting properties depending on their structure, further contributing to their conductivity.
Quantum mechanical effects, high aspect ratio, and the ability to introduce controlled defects or dopants also enhance their electrical conductivity.
The Structure of Carbon Nanotubes
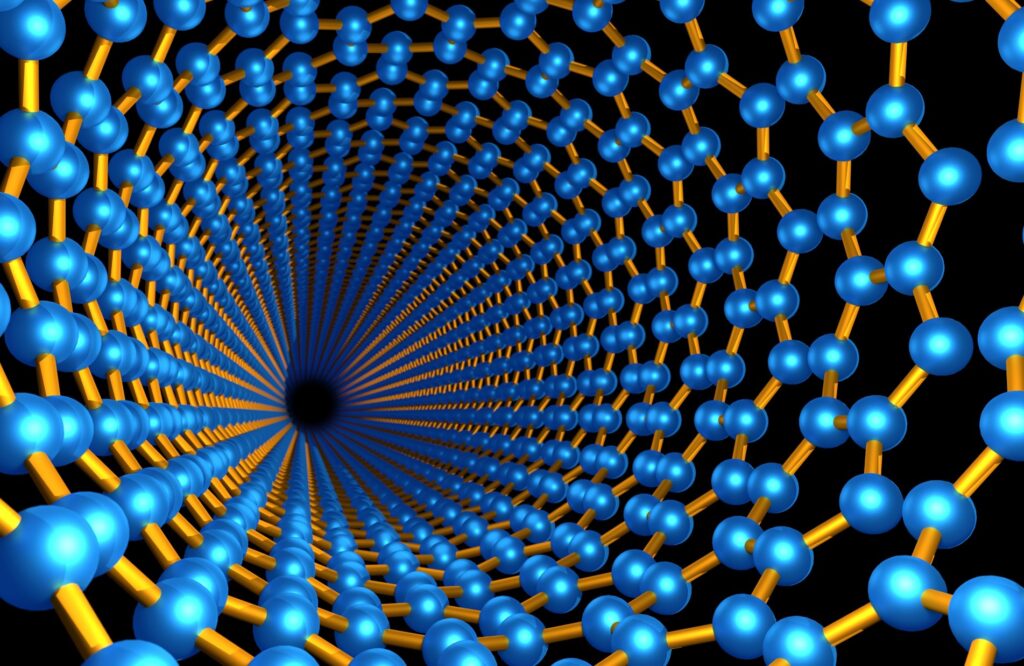
Basic Structure and Formation of CNTs
Hexagonal Lattice Arrangement: The basic structure of carbon nanotubes is characterized by a hexagonal lattice arrangement of carbon atoms. This lattice is rolled into a seamless cylindrical tube, forming the nanotube structure.
Graphene Precursor: Carbon nanotubes are essentially rolled-up graphene sheets, where graphene, a single layer of carbon atoms arranged hexagonally, serves as the precursor.
The properties of carbon nanotubes are significantly influenced by chirality, which refers to the specific arrangement of carbon atoms in the hexagonal lattice.
Chirality influences the electrical, mechanical, and optical characteristics of the nanotube.
Formation Mechanisms: Carbon nanotubes can be formed through various methods, including arc discharge, chemical vapor deposition (CVD), and laser ablation.
These methods involve the use of carbon-containing precursors and specific conditions to encourage the growth of nanotubes.
Different Types of Carbon Nanotubes and Their Unique Properties
Single-Walled Carbon Nanotubes (SWCNTs):
- Properties: SWCNTs exhibit extraordinary electrical conductivity, high tensile strength, and unique optical properties. Their properties are highly dependent on chirality.
Multi-Walled Carbon Nanotubes (MWCNTs):
- Structure: Comprise multiple concentric layers of graphene rolled into tubes.
- Properties: MWCNTs retain some of the remarkable properties of SWCNTs, including high strength and electrical conductivity. The presence of multiple walls adds complexity to their electronic structure.
Armchair, Zigzag, and Chiral Nanotubes:
- Armchair: Chirality where the hexagons form “armchair” patterns. Exhibits metallic properties.
- Zigzag: Chirality with hexagons arranged in a “zigzag” pattern. Can be metallic or semiconducting.
- Chiral: Chirality with a unique arrangement, resulting in a combination of metallic and semiconducting properties. Chirality significantly influences electronic behavior.
Functionalized Carbon Nanotubes:
- Functionalization: Involves modifying the surface of CNTs with functional groups.
- Properties: Functionalized CNTs can exhibit altered chemical, electronic, and mechanical properties, expanding their potential applications in areas such as drug delivery and nanocomposites.
The Quantum Mechanics Behind CNTs
Band Structure and Electron Behavior in CNTs
Band Structure:
- Graphene Band Structure: The band structure of carbon nanotubes is intricately connected to that of graphene, exhibiting distinct energy bands for electrons.
This relationship underscores the unique electronic properties shared between carbon nanotubes and graphene.
Graphene has valence and conduction bands, and the region between them is known as the Dirac cone.
- Quantization of Energy Levels: When graphene is rolled into a tube to form a carbon nanotube, the quantization of energy levels occurs along the circumferential direction.
This quantization leads to the formation of discrete energy bands in carbon nanotubes.
Chirality and Electronic Properties:
- Metallic and Semiconducting Behavior: The chirality of carbon nanotubes significantly influences their electronic properties. Depending on the chirality, carbon nanotubes can exhibit metallic or semiconducting behavior.
- Armchair and Zigzag Nanotubes: Armchair nanotubes tend to be metallic, while zigzag nanotubes can be either metallic or semiconducting, depending on their width.
Quantum Confinement:
- Confinement in One Dimension: Carbon nanotubes exhibit quantum confinement in one dimension due to their tubular structure. This confinement leads to discrete energy levels for electrons along the circumference of the nanotube.
Quantum Tunneling and Its Role in CNT Conductivity
Quantum Tunneling:
- Definition: Quantum tunneling is a quantum mechanical phenomenon where particles, such as electrons, can pass through a potential barrier despite lacking sufficient energy to overcome it classically.
- Role in Conductivity: In carbon nanotubes, quantum tunneling plays a crucial role in their high conductivity.
The discrete energy levels and the quantum nature of electrons allow them to tunnel through potential barriers within the nanotube structure.
Ballistic Conduction:
- Limited Scattering: Quantum tunneling enables ballistic conduction in carbon nanotubes, meaning that electrons can move through the nanotube without significant scattering.
- Efficient Electron Transport: The absence of scattering allows for efficient electron transport, contributing to the high electrical conductivity observed in carbon nanotubes.
Quantum Resistance:
- Quantum of Conductance:
- Due to the quantum characteristics of electron transport, the electrical conductance of carbon nanotubes is quantized in units of 2e^2/h, where e‘ represents the elementary charge, and ‘h‘ is Planck’s constant.
Types of Carbon Nanotube Conductivity
Metallic vs. Semiconducting CNTs
Metallic Carbon Nanotubes (m-CNTs):
- Characteristics: Metallic carbon nanotubes are those that exhibit metallic behavior, meaning they have a high electrical conductivity and a linear relationship between current and voltage.
- Armchair Nanotubes: Armchair nanotubes often display metallic behavior due to their specific arrangement of carbon atoms, which allows for efficient electron transport.
Semiconducting Carbon Nanotubes (s-CNTs):
- Characteristics: Semiconducting carbon nanotubes have an energy band gap, meaning there is a range of energy levels that electrons cannot occupy.
This bandgap gives rise to semiconductor-like behavior, including variable conductivity based on external conditions.
- Zigzag Nanotubes: Zigzag nanotubes can exhibit semiconducting behavior depending on their specific chirality and diameter.
Chirality and Conductivity:
- Chirality Influence: The arrangement of carbon atoms in the hexagonal lattice, or chirality, is crucial in determining whether a carbon nanotube is metallic or semiconducting.
- Armchair, Zigzag, and Chiral Nanotubes: Armchair nanotubes tend to be metallic, zigzag nanotubes can be either metallic or semiconducting, and the chirality of a nanotube influences its electronic properties.
How the Arrangement of Carbon Atoms Affects Conductivity
Chirality-Dependent Conductivity:
- Metallic Nanotubes: The metallic behavior in armchair nanotubes arises from the specific arrangement of carbon atoms that allows for the formation of a metallic band structure.
- Semiconducting Nanotubes: In zigzag or chiral nanotubes, the arrangement of carbon atoms introduces a bandgap, leading to semiconducting behavior.
Band Structure and Energy Gaps:
- Metallic Nanotubes: Metallic nanotubes have a band structure that allows electrons to move freely, contributing to high electrical conductivity.
- Semiconducting Nanotubes: Semiconducting nanotubes have an energy gap that restricts the movement of electrons until sufficient energy is provided to overcome this gap, allowing for tunable conductivity.
Diameter-Dependent Properties:
- Quantum Confinement: The diameter of a carbon nanotube also influences its electronic properties. Smaller-diameter nanotubes may exhibit quantum confinement effects, affecting their conductivity.
- Tubular Structure: The tubular structure of carbon nanotubes, combined with the arrangement of carbon atoms, contributes to efficient electron transport and the observed conductivity.
Applications of Conductive Carbon Nanotubes
Electronics and Nanotechnology
Transistors and Nanoelectronics:
- High Conductivity: The exceptional electrical conductivity of carbon nanotubes makes them promising candidates for high-performance transistors in nanoelectronics.
- Miniaturization: Carbon nanotubes can contribute to the continued miniaturization of electronic components, enabling the development of smaller, more efficient devices.
Interconnects in Integrated Circuits:
- Low Resistance: The low electrical resistance of carbon nanotubes makes them attractive for use as interconnects in integrated circuits.
- Heat Dissipation: Carbon nanotubes also exhibit excellent thermal conductivity, addressing challenges related to heat dissipation in densely packed electronic devices.
Sensors and Detectors:
- Sensitivity: Carbon nanotubes, with their high surface area and responsiveness to environmental changes, are well-suited for diverse sensor applications like gas sensors, biosensors, and environmental sensors
- Biomedical Applications: Carbon nanotube-based sensors show promise in medical diagnostics, detecting biomolecules for applications in healthcare.
Transparent Conductive Films:
- Flexibility and Transparency: Carbon nanotubes can be used to produce transparent conductive films with applications in flexible electronics, touchscreens, and solar cells.
- Conductivity and Light Transmission: The combination of high conductivity and light transmission makes carbon nanotubes an attractive alternative to traditional transparent conductive materials.
Role in Developing Advanced Materials and Devices
Composite Materials:
- Reinforcement: Carbon nanotubes can be incorporated into composite materials, enhancing their mechanical strength and electrical conductivity.
- Lightweight and Strong: Carbon nanotubes’ lightweight and strong nature makes them valuable for aerospace, automotive, and sports equipment applications.
Energy Storage Devices:
- Supercapacitors: Carbon nanotubes are used to develop high-energy and power-density supercapacitors.
- Battery Electrodes: Incorporating carbon nanotubes into battery electrodes can improve conductivity and contribute to the development of advanced energy storage systems.
Conductive Coatings:
- Antistatic Coatings: Carbon nanotubes are employed in antistatic coatings for various materials and devices to prevent the buildup of static electricity.
- Corrosion Resistance: Carbon nanotubes’ conductivity and corrosion-resistant properties make them valuable for protective coatings in harsh environments.
Thermal Management:
- Heat Dissipation: Carbon nanotubes’ high thermal conductivity is exploited for thermal management applications, such as heat sinks and thermal interface materials.
- Thermoelectric Devices: Carbon nanotubes are investigated for use in thermoelectric devices that can convert waste heat into electricity.
Challenges and Innovations
Limitations in CNT Conductivity
Chirality-Dependent Properties:
- Limited Metallic Behavior: While armchair nanotubes exhibit metallic behavior, achieving metallic conductivity in other types of nanotubes, especially those of specific chirality, can be challenging.
- Semiconducting Behavior: Semiconducting nanotubes introduce limitations in certain applications, requiring careful selection or modification to suit the desired conductivity requirements.
Synthesis and Purity:
- Impurities and Defects: Impurities and defects occurring during the synthesis process can adversely affect the electrical conductivity of carbon nanotubes.
- Uniformity: Achieving a high level of uniformity in terms of diameter, chirality, and structure remains a challenge, affecting the consistency of conductivity.
Contact Resistance:
- Electrode Interface: The contact resistance between carbon nanotubes and electrodes can hinder efficient electron transport, affecting the overall conductivity of devices.
- Interface Engineering: Developing effective methods to optimize the interface between carbon nanotubes and electrodes is crucial for overcoming contact resistance challenges.
Scaling-Up Production:
- Cost and Scalability: Large-scale production of high-quality carbon nanotubes at an economically viable cost remains a challenge.
- Industrial Integration: Incorporating carbon nanotubes into established industrial processes for electronics and materials applications presents challenges that must be overcome for widespread adoption.
Ongoing Research and Innovations to Enhance CNT Conductivity
Chirality Engineering:
- Tailoring Properties: Researchers are exploring methods to control and engineer the chirality of carbon nanotubes, aiming to achieve specific electronic properties, including metallic behavior.
- Post-Synthesis Modification: Techniques such as chemical functionalization and post-synthesis sorting are being investigated to modify and separate nanotubes based on chirality.
Defect Engineering:
- Purification Techniques: Advancements in purification techniques, such as selective functionalization and chemical treatments, aim to reduce impurities and defects in carbon nanotubes.
- In Situ Repair: Researchers are exploring in situ methods to repair defects during the synthesis process, enhancing carbon nanotubes’ overall quality and conductivity.
Contact Interface Optimization:
- Nanotube-Electrode Interface Design: Innovations in electrode design and materials focus on optimizing the interface between carbon nanotubes and electrodes to minimize contact resistance.
- Self-Healing Interfaces: Development of self-healing or adaptive materials for electrode interfaces to maintain optimal contact over extended usage.
Advanced Synthesis Techniques:
- Catalyst Design: Catalyst design and control innovations during synthesis aim to produce carbon nanotubes with improved conductivity and uniformity.
- Continuous Production: Advances in continuous production methods seek to address scalability issues, enabling large-scale manufacturing of high-quality carbon nanotubes.
Hybrid Materials:
- Integration with Other Materials: Researchers are exploring the integration of carbon nanotubes with other materials to create hybrid structures that leverage the unique properties of both components.
- Functionalization Techniques: Functionalizing carbon nanotubes with specific molecules or polymers can enhance their properties and broaden their range of applications.
Comparison with Other Materials
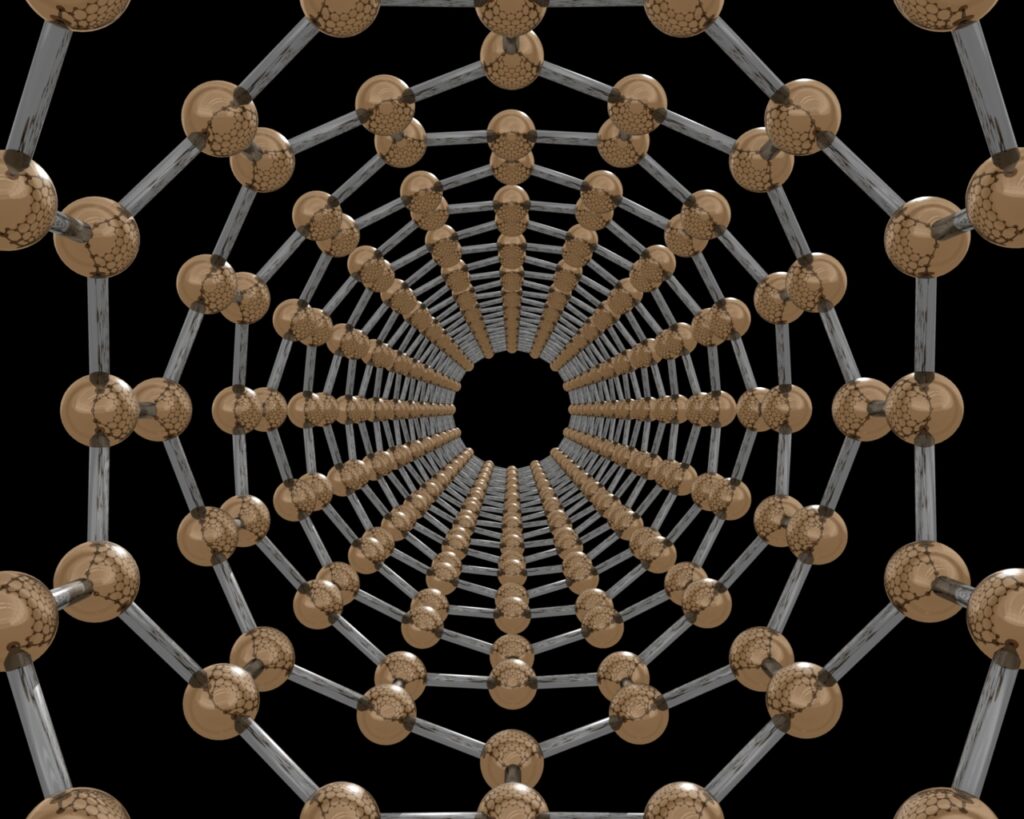
How do CNTs Compare to Traditional Conductive Materials?
Comparison with Copper:
- Electrical Conductivity: Carbon nanotubes (CNTs) can exhibit electrical conductivity comparable to or even surpassing that of copper, a traditional metal known for its excellent conductivity.
- Weight: CNTs are significantly lighter than copper, offering potential advantages in applications where weight is critical.
Comparison with Silicon:
- Semiconducting Properties: Carbon nanotubes can exhibit either metallic or semiconducting behavior, allowing for versatility in electronic applications. Silicon, commonly used in semiconductors, primarily exhibits semiconducting behavior.
- Size and Miniaturization: The nanoscale dimensions of carbon nanotubes enable the miniaturization of electronic components, offering potential advantages over bulkier silicon-based devices.
Comparison with Graphene:
- Structure: Carbon nanotubes and graphene share a hexagonal lattice of carbon atoms, yet their structures differ.
Graphene is a two-dimensional sheet, while carbon nanotubes are one-dimensional cylindrical structures.
- Applications: Both materials have unique properties, but carbon nanotubes and graphene have applications in different areas.
Carbon nanotubes are often used in nanoelectronics and composite materials, while graphene is applied in transparent conductive films and energy storage devices.
Advantages and Disadvantages of Using CNTs for Electrical Conductivity
Advantages:
- High Electrical Conductivity: CNTs exhibit exceptional electrical conductivity, making them suitable for applications in electronics and electrical devices.
- Lightweight: Carbon nanotubes are lightweight, which can be advantageous in applications where weight reduction is crucial, such as aerospace and automotive industries.
- Thermal Conductivity: CNTs also possess excellent thermal conductivity, addressing challenges related to heat dissipation in electronic devices.
- Versatility: The ability of carbon nanotubes to exhibit both metallic and semiconducting behavior, depending on chirality, provides versatility for various electronic applications.
Disadvantages:
- Synthesis Challenges: Achieving high-quality and consistent synthesis of carbon nanotubes remains a challenge, impacting their widespread commercial adoption.
- Chirality Dependency: The properties of carbon nanotubes, particularly whether they exhibit metallic or semiconducting behavior, are strongly dependent on chirality, which can complicate their use in specific applications.
- Cost: Large-scale production of high-quality carbon nanotubes can be expensive, limiting their competitiveness with traditional materials in some applications.
FAQs
What gives carbon nanotubes their unique electrical conductivity?
Carbon nanotubes exhibit high electrical conductivity due to their sp2 hybridized carbon atoms, forming a seamless hexagonal lattice that allows electrons to move freely.
How does the structure of carbon nanotubes contribute to conductivity?
Carbon nanotubes’ one-dimensional structure minimizes the electrons’ scattering, promoting efficient electron transport and high conductivity.
What role do carbon nanotube walls play in conductivity?
Single-walled carbon nanotubes (SWCNTs) demonstrate superior conductivity compared to multi-walled carbon nanotubes (MWCNTs) due to fewer defects and a more regular structure.
Can metallic and semiconducting carbon nanotubes conduct electricity differently?
Yes, metallic carbon nanotubes exhibit higher electrical conductivity than semiconducting ones, as metals allow for more efficient electron transport.
How does the chirality of carbon nanotubes affect conductivity?
The chirality (wrapping pattern) influences whether a carbon nanotube is metallic or semiconducting, impacting its electrical properties.
Do defects in carbon nanotubes affect their conductivity?
Defects, such as vacancies or impurities, can hinder conductivity by scattering electrons, but careful synthesis methods aim to minimize these defects.
Why are carbon nanotubes considered excellent conductors in nanoscale electronics?
Carbon nanotubes’ small dimensions and unique electrical properties make them promising candidates for nanoscale electronic devices, offering high conductivity and efficiency.
Can carbon nanotubes be used as interconnects in electronic devices?
Yes, carbon nanotubes are explored as potential interconnects in electronic circuits due to their excellent electrical conductivity and nanoscale dimensions.
How do environmental factors impact the conductivity of carbon nanotubes?
Environmental factors such as humidity and gas exposure can introduce additional charge carriers or alter the surface properties, influencing the electrical conductivity of carbon nanotubes.
Can carbon nanotubes be functionalized to enhance their conductivity?
Yes, functionalization with certain chemical groups can modify the electronic properties of carbon nanotubes, potentially enhancing their conductivity or introducing specific functionalities.
Conclusion
In conclusion, carbon nanotubes (CNTs) stand at the forefront of materials science, exhibiting remarkable electrical conductivity and unique properties that differentiate them from traditional conductive materials.
Their one-dimensional structure, tunable electronic behavior, and exceptional thermal conductivity make them promising candidates for electronics, nanotechnology, and advanced materials applications.
Despite facing challenges such as chirality dependency and synthesis issues, ongoing research and innovations strive to harness the full potential of CNTs, paving the way for their integration into a wide range of industries and technologies.
As these challenges are tackled, carbon nanotubes stand ready to play a transformative role in shaping the future of electronic devices, materials, and technological advancements.